Enhanced Resolution for Longer Oligonucleotide Analytes With a MaxPeak™ Premier Oligonucleotide BEH C18 300 Å Column
Abstract
Ion-pairing reversed phase liquid chromatography is widely used to characterize the identity, purity, and integrity of oligonucleotides and this is important to the development and quality control of both diagnostics and therapeutic medicines. Detection sensitivity and retention of these polynucleotide analytes not only depend on the surface chemistry but also pore size of the column stationary phase. The selection of an appropriate pore size can enable longer oligonucleotides to efficiently interact with stationary phase and minimize restricted diffusion effects such that improved peak capacities can be achieved. Here, we report the efficient separation of single stranded (ss) heteromeric deoxyribonucleotide (DNA) ladders on an ACQUITY™ Premier Oligonucleotide BEH C18 300 Å 1.7 µm Column in a manner that is compatible with online mass spectrometric (MS) detection.
Benefits
- New reference materials for oligonucleotide LC available as ssDNA oligonucleotide ladders
- Excellent resolution of long-mer oligonucleotides with an ACQUITY Premier Oligonucleotide BEH C18 300 Å Column
- Improved resolution for oligonucleotides exceeding 70 nucleotides while maintaining performance for shorter oligonucleotides
- Dependable and consistent separation performance independent of the applied ion-pairing reagent
- Reproducible high-quality performance and confident mass measurements
Introduction
Advanced analytical tools are helping to accelerate the development of novel nucleic acid-based diagnostics and therapeutics.1–4 Reversed phase liquid chromatography coupled with mass spectrometry (RPLC-MS) is a preferred choice for qualitative and quantitative characterization of the oligonucleotides during various stages of development and pharmacokinetic studies. Volatile alkylamines acting as ion-pairing (IP) reagents improve the retention and resolution of oligonucleotides on reversed phase column during these types of analyses.5–7 The type of IP reagent determines separation selectivity and retention during IP-RPLC.3 Moreover, buffering IP reagents with weak acids like hexafluoroisopropanol makes oligonucleotide IP-RPLC analysis compatible with mass spectrometry (MS).8–10
Apart from the surface chemistry of a stationary phase, it is important to consider its pore size. The pore size of a stationary phase can affect the selectivity, retention, and resolution of an oligonucleotide separation. Average pore size determines whether an analyte molecule can diffuse into and out of the porous particle structure and partition between the inter- and intraparticle spaces.11 Smaller pore size (90–130 Å) stationary phases provide larger surface areas per bed length and correspondingly longer retention times for shorter oligonucleotides (<50mer) compared to wider pore size stationary phases (300 Å) with relatively smaller surface area.12 However, larger analytes encounter steric hindrance when traversing smaller pore structures. This can be seen when analytes are restricted from accessing intraparticle surface areas or show peak broadening from impeding diffusion out of a small pore. In contrast, the wider pore increases the accessibility to longer oligonucleotides and improve the resolution.4,12 Here, we present consistently improved resolution and high-quality performance for oligonucleotides above 70mer including 80, 90, and 100mers on a 300 Å pore size premier BEH C18 Column compared to the smaller pore size column.
Experimental
Sample Information
Reference materials were acquired from Waters (Milford, MA). The ssDNA 10 to 60 Ladder (Waters p/n: 186009449) and ssDNA 20 to 100 Ladder (Waters p/n: 186009448) (Table 1) were individually resuspended in 100 µL of Milli-Q water. After gentle mixing, the vial contents were transferred to a 300 µL capacity polypropylene autosampler vial (p/n: 186002639) for ion-pairing reversed phase liquid chromatography (IP-RP-LC) mass spectrometry (MS) analysis in negative ion mode using the BioAccord™ RDa detector.
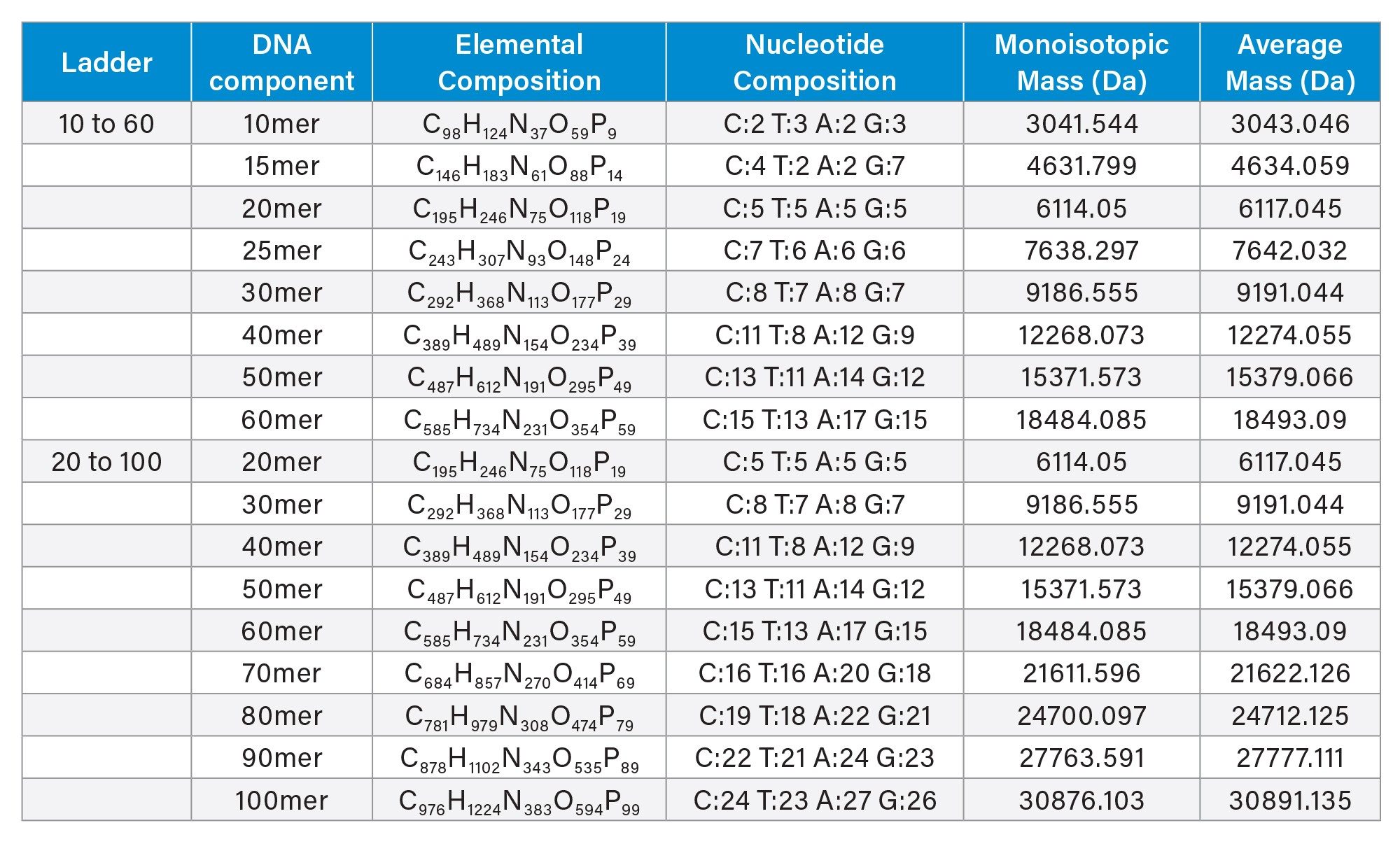
LC Conditions 1
LC system: |
ACQUITY Premier BSM System (part of BioAccord System) |
Detector: |
ACQUITY UPLC TUV Detector |
Wavelength: |
260 nm |
Columns: |
ACQUITY Premier Oligonucleotide BEH C18, 300 Å, 1.7 µm, 2.1 X 150 mm, p/n: 186010541 ACQUITY Premier Oligonucleotide BEH C18, 130 Å, 1.7 µm, 2.1 X 150 mm, p/n: 186009486 |
Column temperature: |
70 °C |
Sample temperature: |
4 °C |
Injection: |
5 μL |
Flow rate: |
0.3 mL/min |
Mobile phase A: |
7 mM Triethylamine (TEA) as the IP reagent and 40 mM 1,1,1,3,3,3-hexafluoroisopropanol (HFIP) in Milli-Q water, pH 8.6 |
Mobile phase B: |
3.5 mM TEA and 20 mM HFIP in 50:50 Methanol: Milli-Q water |
Gradient Table 1
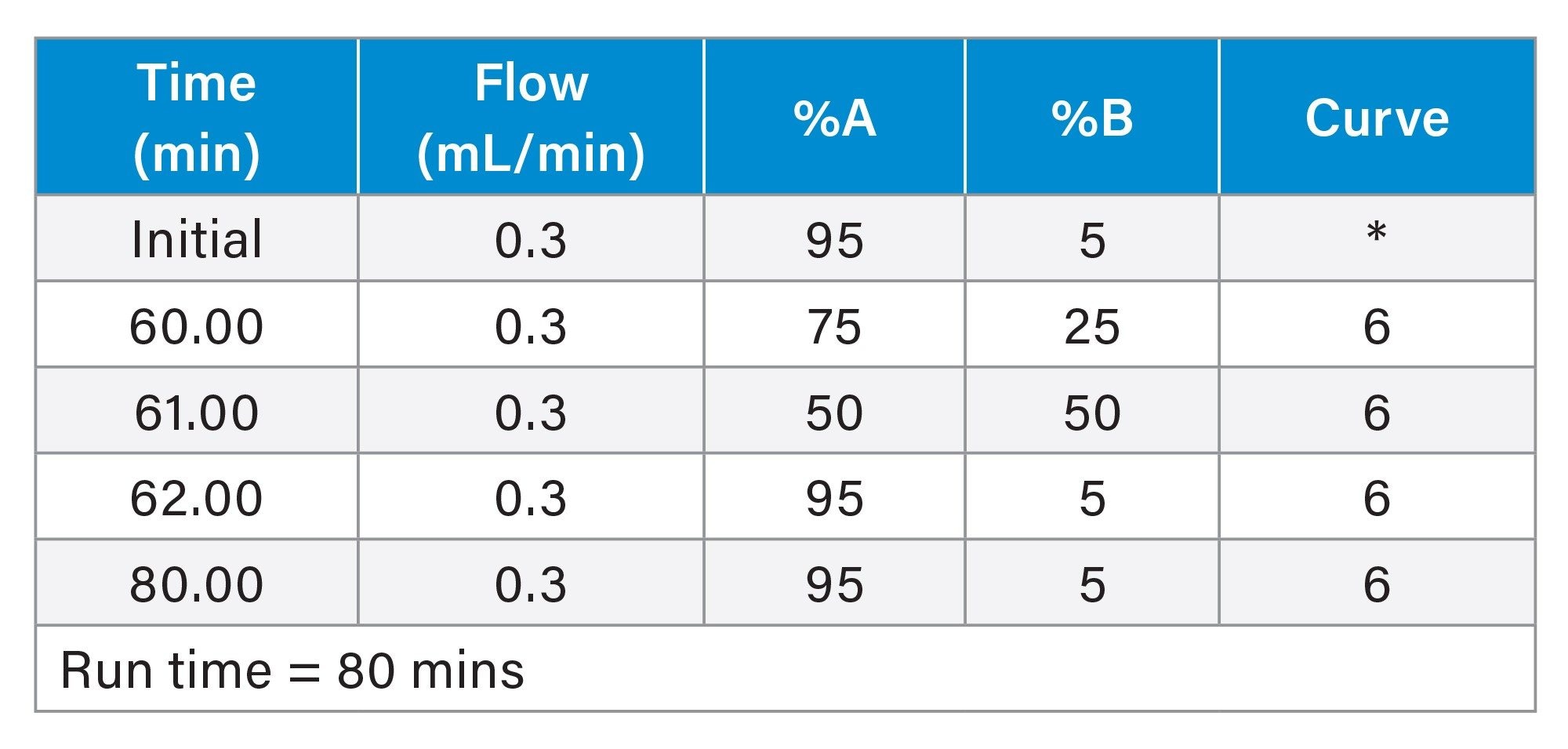
LC Conditions 2
LC system: |
ACQUITY Premier BSM System (part of BioAccord System) |
Detector: |
ACQUITY UPLC TUV Detector |
Wavelength: |
260 nm |
Columns: |
ACQUITY Premier Oligonucleotide BEH C18, 300 Å, 1.7µm, 2.1 X 150 mm ACQUITY Premier Oligonucleotide BEH C18, 130 Å, 1.7µm, 2.1 X 150 mm |
Column temperature: |
70 °C |
Sample temperature: |
4 °C |
Injection: |
5 μL |
Flow rate: |
0.4 mL/min |
Mobile phase A: |
0.1% N,N-diisopropylethylamine (DIPEA) as the IP reagent and 1% 1,1,1,3,3,3-hexafluoroisopropanol (HFIP) in Milli-Q water |
Mobile phase B: |
0.0375% DIPEA and 0.075% HFIP in 65:35 Acetonitrile: Milli-Q water |
Gradient Table 2
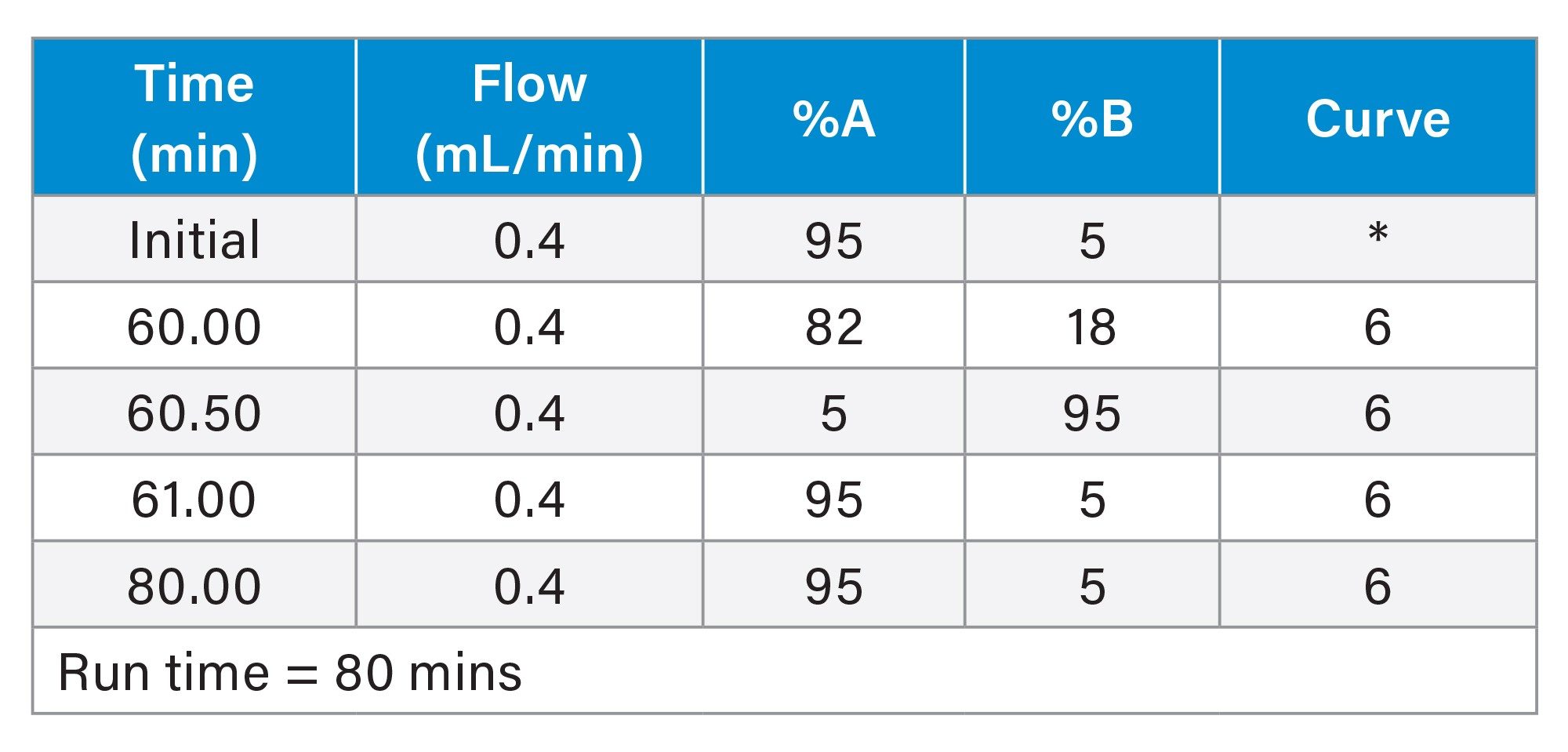
MS Conditions
MS system: |
BioAccord LC-MS System |
||
Detector: |
ACQUITY RDa Detector |
||
Mode: |
Full scan with fragmentation |
||
Polarity: |
Negative |
||
Cone voltage: |
40 V |
||
Fragmentation cone voltage: |
80–200 V |
||
Mass range: |
High (400–5000 m/z) |
||
Scan rate: |
2 Hz |
||
Capillary voltage: |
0.80 kV |
||
Desolvation temperature: |
400 °C |
Informatics
The Intact Mass analysis application of the waters_connect™ platform was employed to compute the neutral mass values of oligonucleotides.
Results and Discussion
The ssDNA 10 to 60 and 20 to 100 Ladders were analyzed by IP-RP-LC-MS using a set of ACQUITY Premier Oligonucleotide BEH C18 Columns containing 300 Å or 130 Å pore size sorbents. Data were acquired in triplicate in negative ion mode, where the cone voltage of electrospray source was alternated between low energy (40 V) and high energy (80–200 V) to acquire the mass values of intact and fragmented oligonucleotides.
Improved resolution of oligonucleotide analytes using a 300 Å pore size BEH C18 sorbent is illustrated in Figures 1–2. Figure 1 shows an overlay of the LC-UV chromatograms obtained with both the ladders using a triethylamine (TEA)-HFIP mobile phase system. Although these columns with different pore size sorbents exhibit similar chromatographic resolution up to 60mer, the wide pore 300 Å column exhibited marked improvement of the separation for 70, 80, 90, and 100mers compared to 130 Å pore size column. The latter column resolved 70 and 80mer as one set of peaks compared to the 90 and 100mer peak set, where the individual components of the pair are only partially resolved. However, both columns yielded the expected number of oligonucleotide peaks, eight for 10 to 60 ladder and nine for 20 to 100 ladder. Importantly, the resolution of shorter oligonucleotides is also maintained without any compromise due to the use of the wider pore column. Interestingly, the identical oligonucleotide standards (20, 30, 40, 50, and 60mer) present in both ladders exhibited near identical retention times even though each ladder was injected independently. This type of reproducible behavior is highly suited to the detection of identical analyte/s in multiple samples, as is encountered in oligonucleotide therapeutic development, DMPK studies and bioanalysis. The oligonucleotide component observed in each chromatographic peak was confirmed by the acquired electrospray MS spectra (data not shown). As predicted, the 130 Å pore size column produced longer retention times compared to wider pore sorbent column, presumably due to higher pore surface area as proposed in other studies.12

Figure 2 depicts the chromatographic profiles of 10 to 60mer and 20 to 100mer ladders following IP-RP-LC-MS on the 300 Å pore size column using a diisopropylethylamine (DIPEA)-HFIP mobile phase. This mobile phase system exhibited slightly improved resolution for 70, 80, 90, and 100mer DNA ladder components. This indicates robust separation performance for more than one mobile phase system. The slightly enhanced chromatographic resolution that came with the use of DIPEA made it possible to achieve even higher quality MS data.13
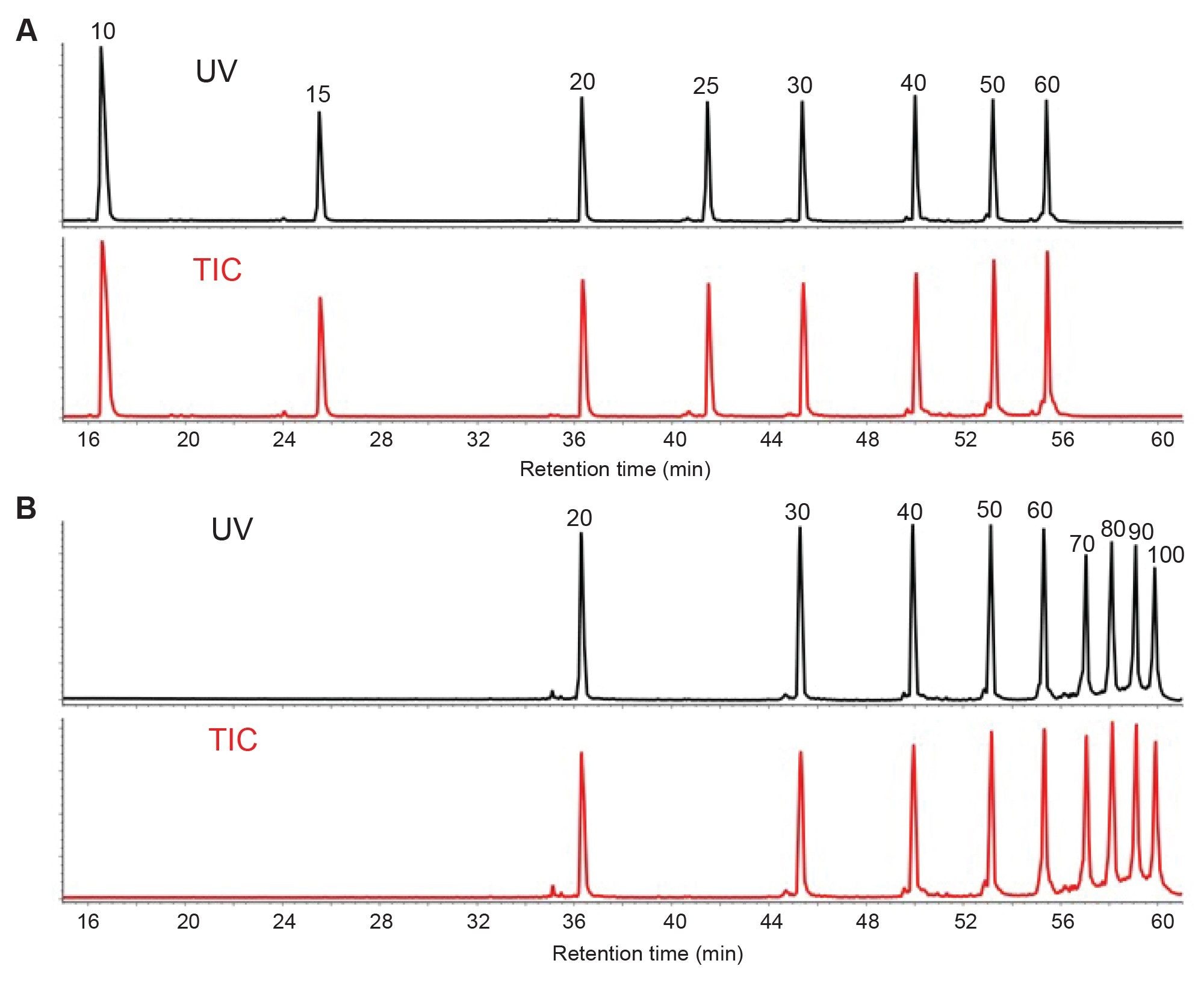
The mass measurements of the resolved oligonucleotide peaks are illustrated in Figure 3 and Table 2. Figure 3 shows the electrospray ion series of the mass spectra obtained for the chromatographic peaks corresponding to 90 (59.12 min, MW 27,778) and 100mer (59.90 min, MW 30,892) ssDNA ladder components. Intact mass analysis of each spectra revealed neutral mass values that correspond well (mass error of <60 ppm) with the expected average mass values.
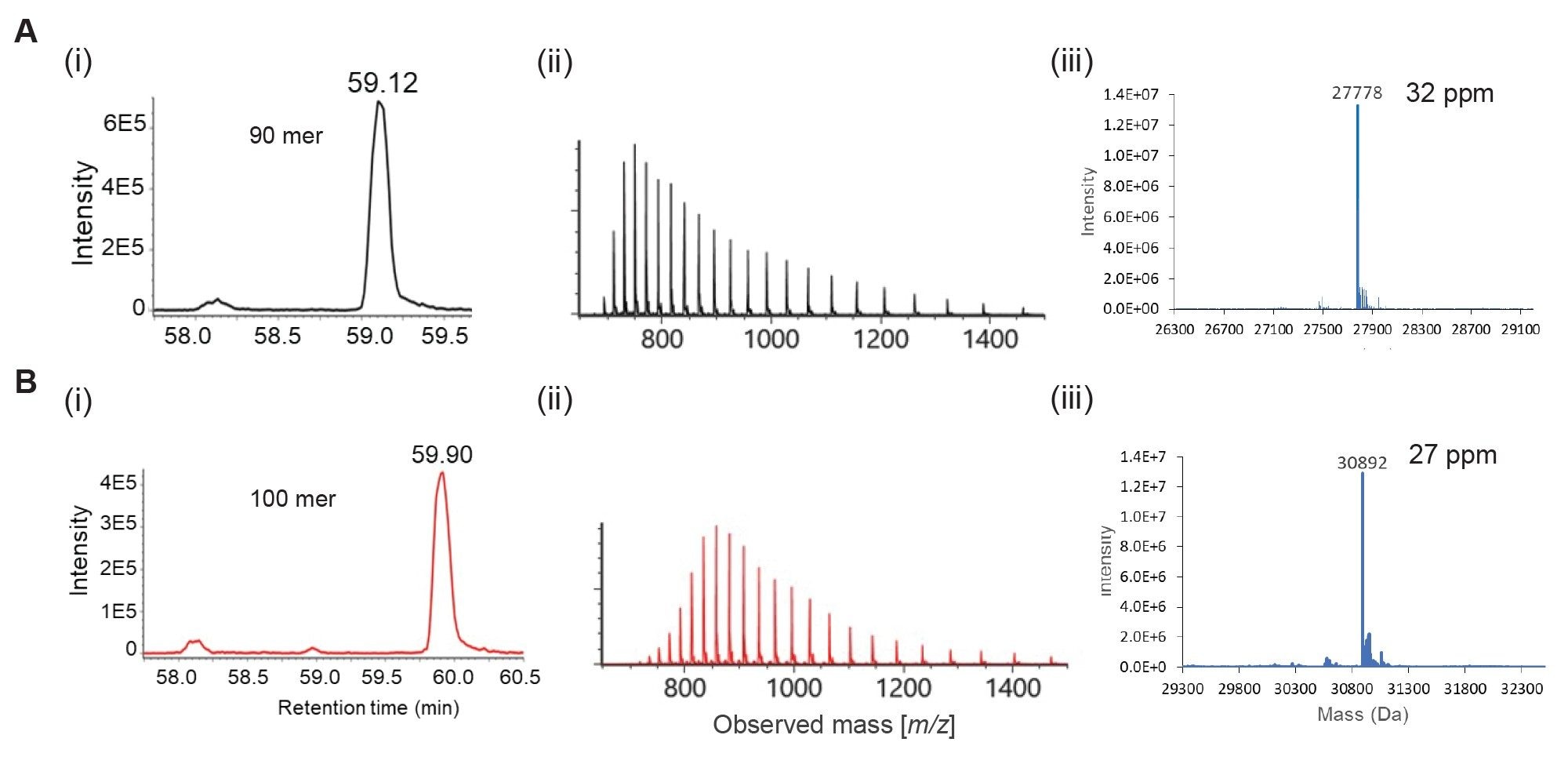
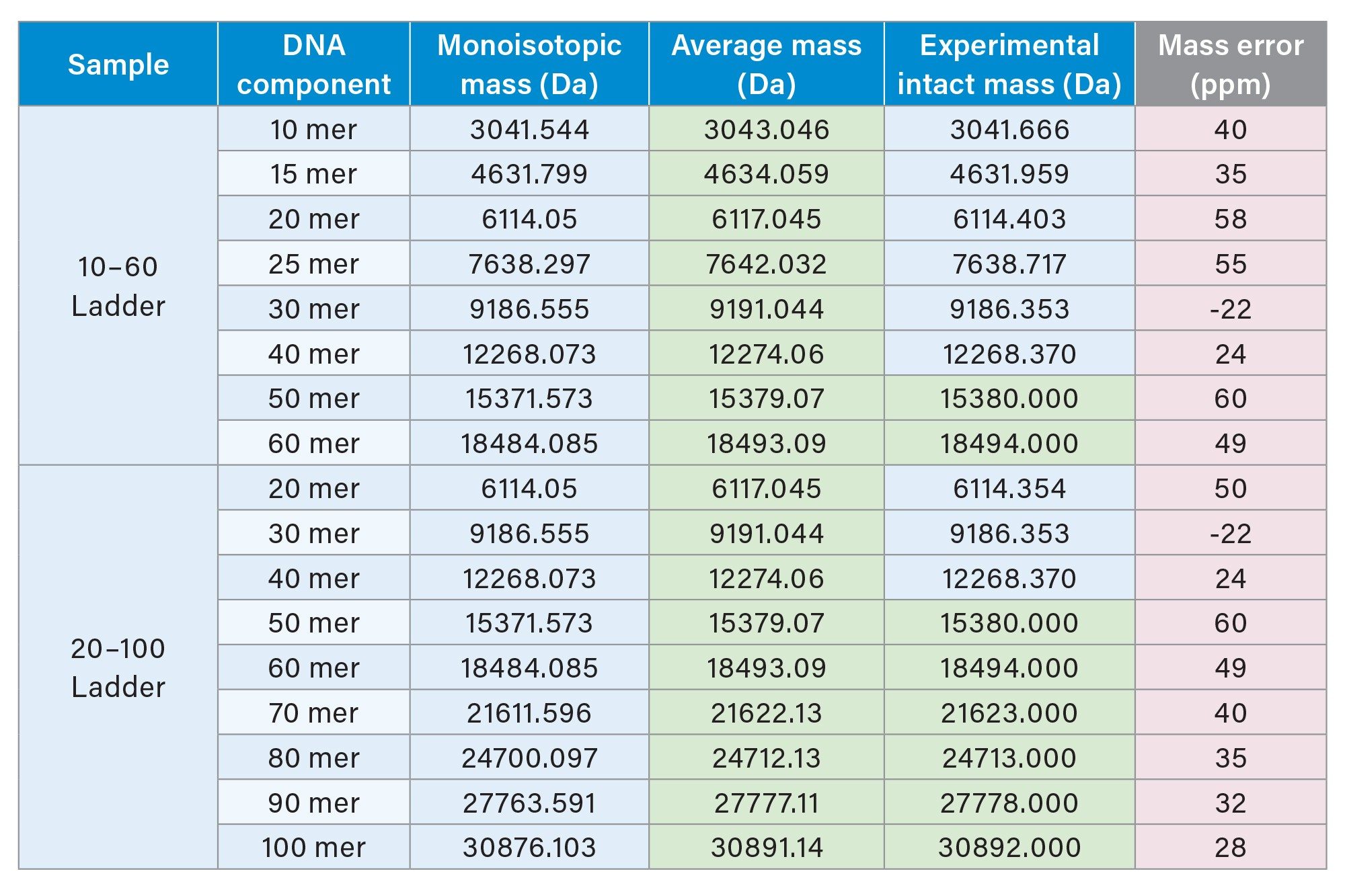
Table 2 depicts the intact mass values of the individual oligonucleotides following deconvolution of the mass spectra by the Intact Mass application of the waters_connect platform. Examination of these deconvoluted mass values indicated higher propensity to match with the monoisotopic mass for oligonucleotides up to 40mer (MW 12,268) and average mass at above 50mer DNA (MW 15,380). This observation confirms that the BioAccord LC-MS System and its RDa mass spectrometer tends to produce a mass resolution of approximately 10,000.
Finally, UV detector response was assessed and found to be highly reproducible when examined from three independent injections of each ladder where the RSD values were <3% (Figure 4).
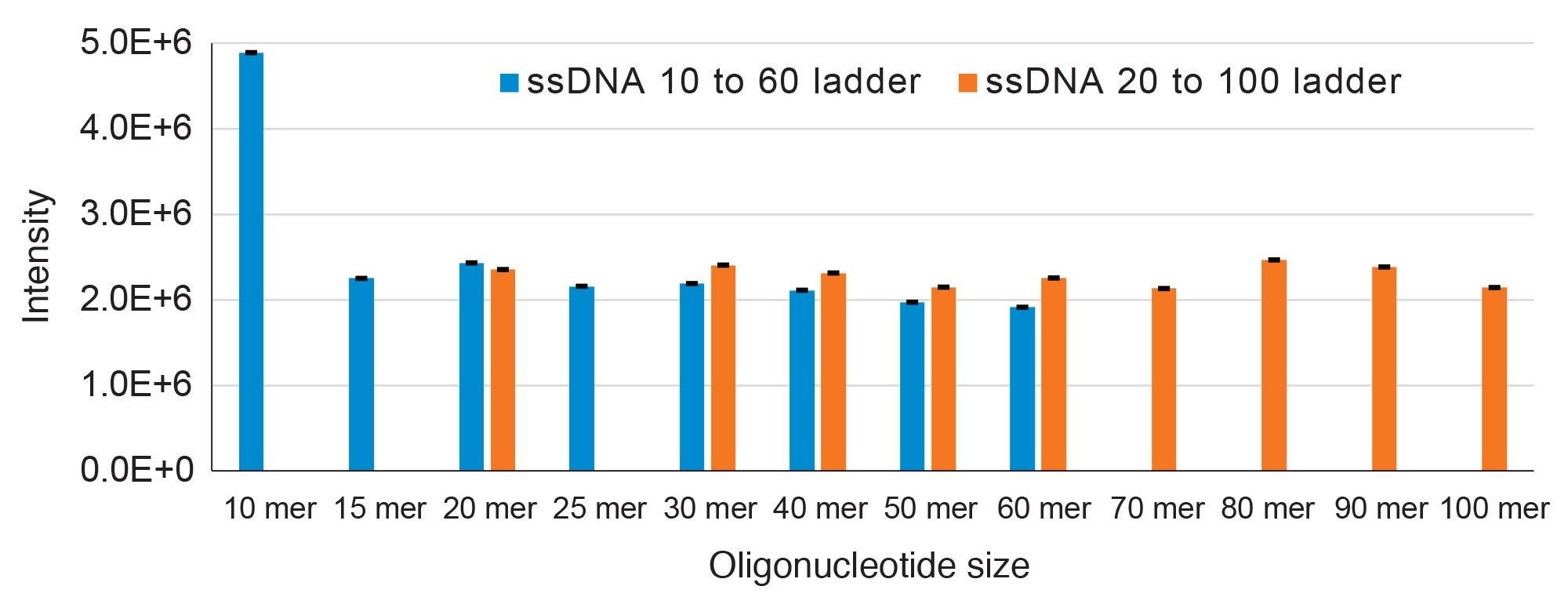
Conclusion
The ACQUITY Premier Oligonucleotide BEH C18, 300 Å Column provides improved resolution for longer oligonucleotide analytes while maintaining excellent resolution of shorter oligonucleotides. The availability of two new LC-Certified reference materials made it possible to quickly confirm the suitability of this type of column with two different ion pairing mobile phase systems. Oligonucleotides of the same size and nucleotide composition exhibited identical retention times irrespective of the sample origin, indicating that these methods are highly suitable for peak tracking and peak ID applications. Ultimately, the combination of high peak capacity column described here and the compliance ready BioAccord LC-MS System has made it significantly easier to achieve in depth analyses of oligonucleotides (and potentially beyond) of 100 nucleotides in length.
References
- Goyon, A., P. Yehl, and K. Zhang, Characterization of Therapeutic Oligonucleotides by Liquid Chromatography. Journal of Pharmaceutical and Biomedical Analysis, 2020. 182: p. 113105.
- Demelenne, A., et al., Analytical Techniques Currently Used in the Pharmaceutical Industry for the Quality Control of RNA-Based Therapeutics and Ongoing Developments. Journal of Chromatography A, 2021. 1651: p. 462283.
- Donegan, M., J.M. Nguyen, and M. Gilar, Effect of Ion-Pairing Reagent Hydrophobicity on Liquid Chromatography and Mass Spectrometry Analysis of Oligonucleotides. Journal of Chromatography A, 2022. 1666: p. 462860.
- Fornstedt, T. and M. Enmark, Separation of Therapeutic Oligonucleotides Using Ion-Pair Reversed-Phase Chromatography Based on Fundamental Separation Science. Journal of Chromatography Open, 2023. 3: p. 100079.
- Apffel, A., et al., New Procedure for the Use of High-Performance Liquid Chromatography–Electrospray Ionization Mass Spectrometry for the Analysis of Nucleotides and Oligonucleotides. Journal of Chromatography A, 1997. 777(1): p. 3–21.
- Gilar, M., et al., Ion-Pair Reversed-Phase High-Performance Liquid Chromatography Analysis of Oligonucleotides: Retention Prediction. Journal of Chromatography A, 2002. 958(1): p. 167–182.
- Huber, C.G., P.J. Oefner, and G.K. Bonn, High-Resolution Liquid Chromatography of Oligonucleotides on Nonporous Alkylated Styrene-Divinylbenzene Copolymers. Analytical Biochemistry, 1993. 212(2): p. 351–358.
- Martin Gilar, K.J.F., Yeva Budman, Jeffrey L. Holyoke, Hamid Davoudi, and John C. Gebler, Characterization of Therapeutic Oligonucleotides Using Liquid Chromatography With On-Line Mass Spectrometry Detection. Oligonucleotides, 2003. 13(4): p. 229–243.
- Erb, R. and H. Oberacher, Comparison of Mobile-Phase Systems Commonly Applied in Liquid Chromatography-Mass Spectrometry of Nucleic Acids. ELECTROPHORESIS, 2014. 35(9): p. 1226–1235.
- McGinnis, A.C., E.C. Grubb, and M.G. Bartlett, Systematic Optimization of Ion-Pairing Agents and Hexafluoroisopropanol for Enhanced Electrospray Ionization Mass Spectrometry of Oligonucleotides. Rapid Communications in Mass Spectrometry, 2013. 27(23): p. 2655–2664.
- Wang, Y., et al., A Theoretical Study of the Separation Principle in Size Exclusion Chromatography. Macromolecules, 2010. 43(3): p. 1651–1659.
- Bagge, J., et al., Impact of Stationary-Phase Pore Size on Chromatographic Performance Using Oligonucleotide Separation as a Model. Journal of Chromatography A, 2020. 1634: p. 461653.
- Croley, T.R., et al., The Chromatographic Role in High Resolution Mass Spectrometry for Non-targeted Analysis. Journal of The American Society for Mass Spectrometry, 2012. 23(9): p. 1569–1578.
720007926, May 2023