Use of Nitrogen as Carrier Gas for GC-MS/MS With Atmospheric Pressure Ionization (APGC) for the Determination of Pesticide Residues in Food
Abstract
Gas chromatography coupled to Tandem Quadrupole Mass Spectrometry (GC-MS/MS) is a powerful analytical technique used for the detection and quantification of pesticide residues in food and environmental samples. This technique historically utilizes helium as a carrier gas. In recent year there have been difficulties sourcing helium worldwide, leading to significant increases in price and difficulties in availability. This has created an increased level of interest for alternative carrier gases for gas chromatography in general. Nitrogen is readily available, relatively inexpensive, and safe compared to other options. The following application demonstrates the ease of transferring GC-MS/MS methods, utilizing an atmospheric pressure ionization source (APGC™ technology) using helium carrier gas to nitrogen carrier gas. APGC achieves improved selectivity and sensitivity when compared to electron ionization (EI) analysis, with APGC demonstrating equivalent performance when using helium or nitrogen as carrier.
A routine method of over 200 pesticides was evaluated on the APGC Xevo™ TQ-S micro System using both carrier gases was performed on a cucumber extract Sample preparation utilized a modified Quick, Easy, Cheap, Effective, Rugged, and Safe (QuEChERS) method. Extracts of infant food (cottage pie) were also prepared for pesticide analysis using nitrogen as the carrier to demonstrate equivalent performance of each gas when using APGC, simply by using a smaller diameter column and scaling the analytical method gas flow parameters.
Benefits
Introduction
Helium is the most commonly used carrier gas for Gas Chromatography.1 Helium’s usage is based on historical applications and instrumentation design, and it was generally assumed to be the preferred carrier gas when its use was not as restricted and/or costly as it is today. Helium is a byproduct of natural gas extraction, and certain helium-producing regions have experienced political instability, resulting in disruptions to the global supply chain. Oil and gas production fluctuations also impact the availability of helium as it primarily sourced as a by-product of these processes. Helium is also used for other technologies such as magnetic resonance imaging (MRI) machines, semiconductor manufacturing, and superconductivity research, further increasing demand. Additionally, some of these applications areas, most notably medical diagnosis, are prioritized over chromatographic applications. This limited supply-demand balance has led to price escalation.2
The advantages of using APGC-MS/MS for pesticide analysis can be found here:
https://www.waters.com/webassets/cms/library/docs/720007815en.pdf
https://www.waters.com/content/dam/waters/en/app-notes/2022/720007708/720007708-en.pdf
https://www.waters.com/content/dam/waters/en/app-notes/2022/720007654/720007654-en.pdf
Helium (He) is becoming a costly choice for a carrier gas for gas chromatography-mass spectrometry (GC-MS) as supplies have been restricted resulting in shortages. Chromatographers have found that He is much more expensive and is not always available when needed. Many have considered switching from He to hydrogen as an alternative. However, there are perceived concerns about its safety, reactivity on older instruments, reduced performance compared to He, and the length and cost of the transition process. This all combines to make the case for using nitrogen. Nitrogen is an inert, renewable gas which is relatively inexpensive compared to the alternatives. Nitrogen already has its use in non-MS detectors but is met with trepidation in MS laboratories due to vacuum source flow requirements. With APGC there is no need for a high vacuum and ionization takes place around a corona pin which induces a nitrogen plasma, so using nitrogen as a carrier gas will have no adverse effects. The mechanism of the APGC technique is gas-phase ion molecule reactions by charge or proton transfer For pesticide residue analysis, source conditions are adjusted to have just enough water present in the ionization chamber to give sufficient sensitivity for compounds that prefer either charge or proton transfer. The instrument setup has very dry conditions, achieved by using appropriate quality nitrogen gas and filtration. Water is then added vials in a holding tray which is placed within the source enclosure.
The objective of this study is to demonstrate the ease of which one can switch to using nitrogen as carrier gas for APGC-MS/MS without having to sacrifice on performance.
Experimental
Sample Description
A QuEChERS extraction was performed on samples of cucumber and infant food (cottage pie) to make matrices in acetonitrile for bracketed calibrations of 203 GC-amenable pesticides (RESTEK p/n: 32562). Both matrices were extracted using a modified version of the QuEChERS CEN method.4 For infant food this also involves SPE clean up by passing through an Oasis™ PRIME HLB cartridge. Matrix matched standards were made over the range of 0.00025 to 0.02 mg/kg and analyzed using the APGC Xevo TQ-S micro System, changing from helium to nitrogen carrier on multiple occasions.
Method Conditions
The changeover process to nitrogen carrier is simply a case of switching the gas supply over and with the appropriate filters even a standard pressurized cylinder of nitrogen can be used. Nitrogen has a lower optimum linear velocity (10–15 cm/s) compared to helium (25–35 cm/s) thus a lower column flow must be used for nitrogen carrier. Therefore, in order to achieve equivalent retention times, the GC method needs to be translated to a smaller length column with a matching phase ratio as the column used for helium analysis. There are tools available online to help with these transitions, the example shown in Figure 1 was used for this analysis .
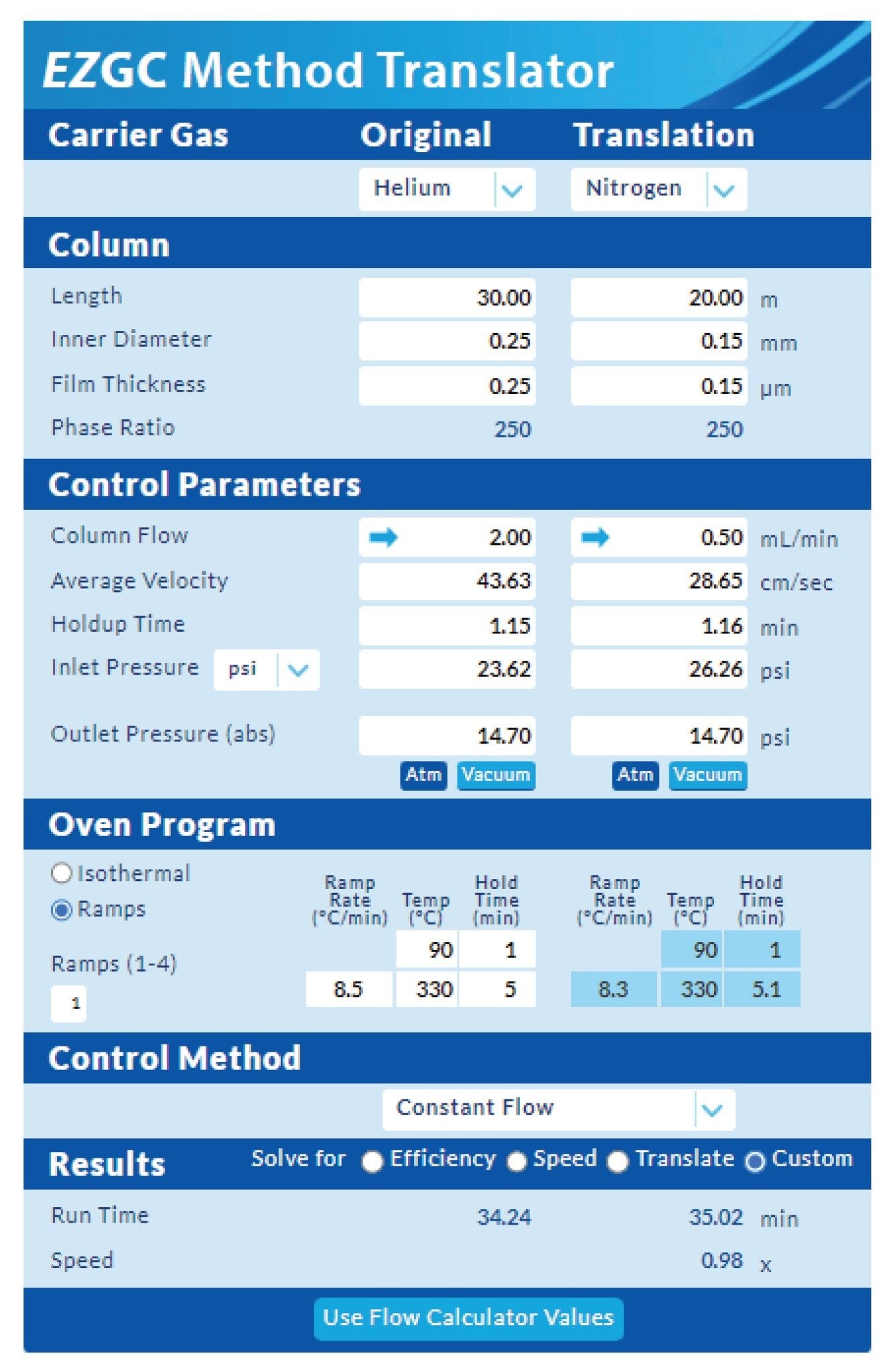
By entering the method parameters for helium the translator will try to match the ‘Holdup Time’ for the nitrogen method and will give a reasonable starting point for a new method, a little method development work may be required with the column flow to match retention times exactly. After initial method development, a solvent focusing step was added to the oven ramp for the nitrogen method for some of early eluting compounds.
GC Conditions
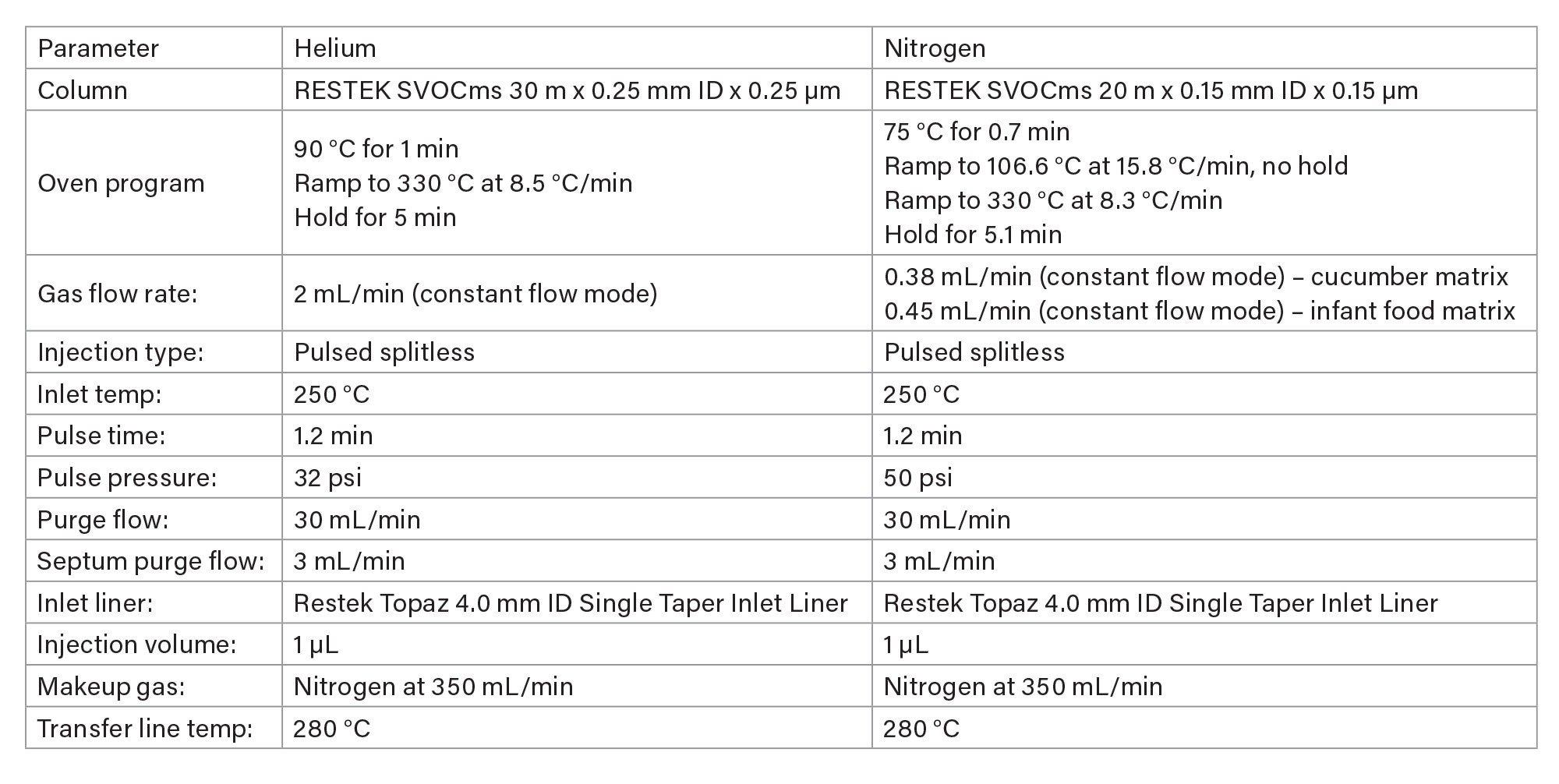
Results and Discussion
Method Translation
Due to nitrogen having a lower optimum linear velocity than helium, the gas flow rate is much lower for the nitrogen method, so a smaller column length column is needed otherwise retention times could be twice as long. Matching the phase ratio of the column to the one used for helium analysis ensures chromatographic performance is retained.
The switch of carrier gas was an easy transition as scaling the column dimensions along with a small amount of method development allows for the retention times to be matched from one carrier gas to another. This allowed many of the method files used for data processing to be kept the same for each type of carrier gas. The nitrogen analysis showed equivalent calibration coefficients for the historically “difficult” compounds as well as equivalent resolution of critical pairs. Both carrier gases analyzed on APGC Xevo TQ-S micro show superior sensitivity to an EI based system and demonstrated consistent performance when using either helium or nitrogen as carrier.
Chromatographic Performance
Figure 2 shows a comparison of early eluting peaks in the infant food matrix. Using the parameters calculated in the method translator it can be shown that peaks will lie within the ±0.2 min range that means the same processing file can be used.
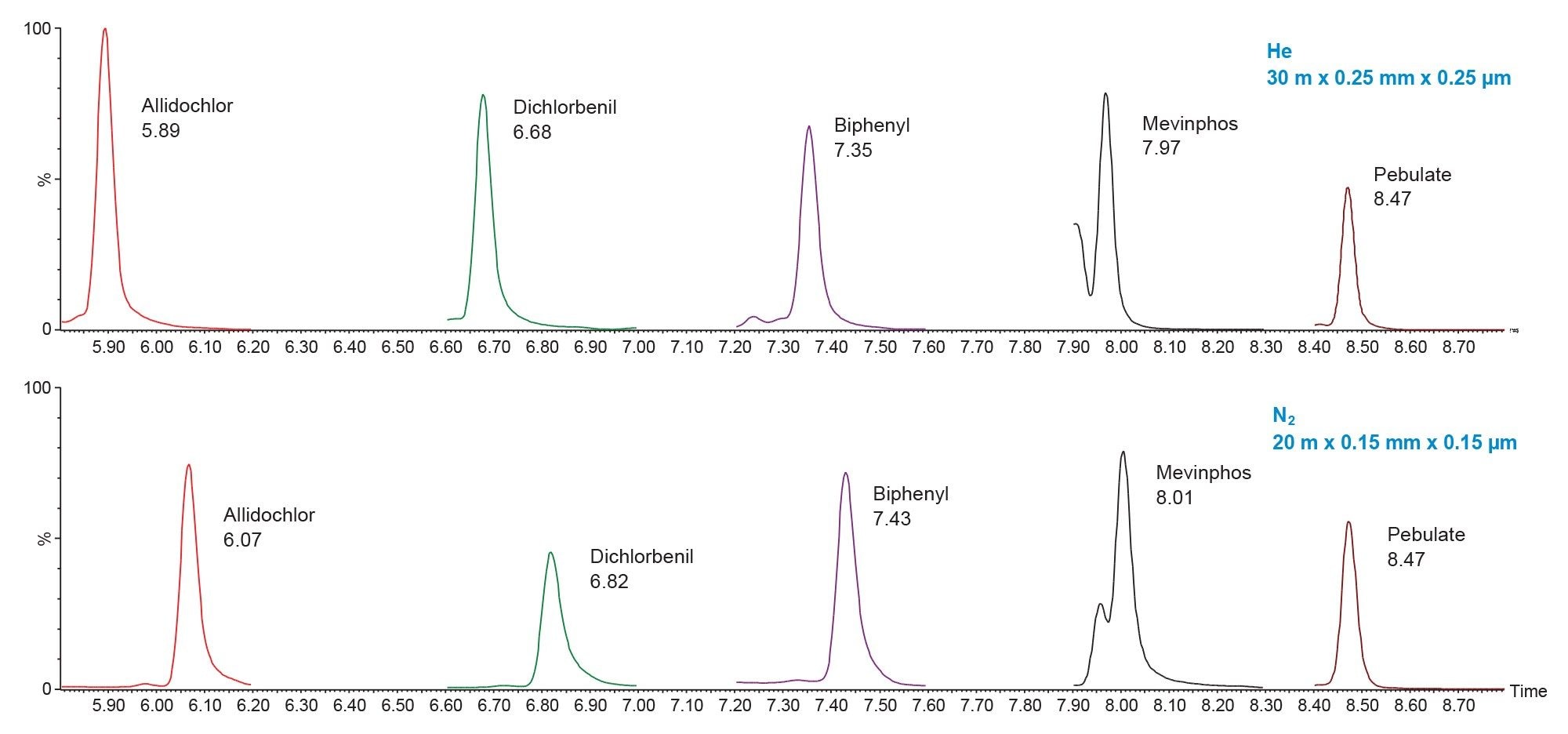
Figure 3 shows late eluting peaks from the cucumber matrix, given more time to develop the method and experiment with column flow, the retention times matched up perfectly. This allowed for the same processing method to be used for each carrier gas across both matrices.
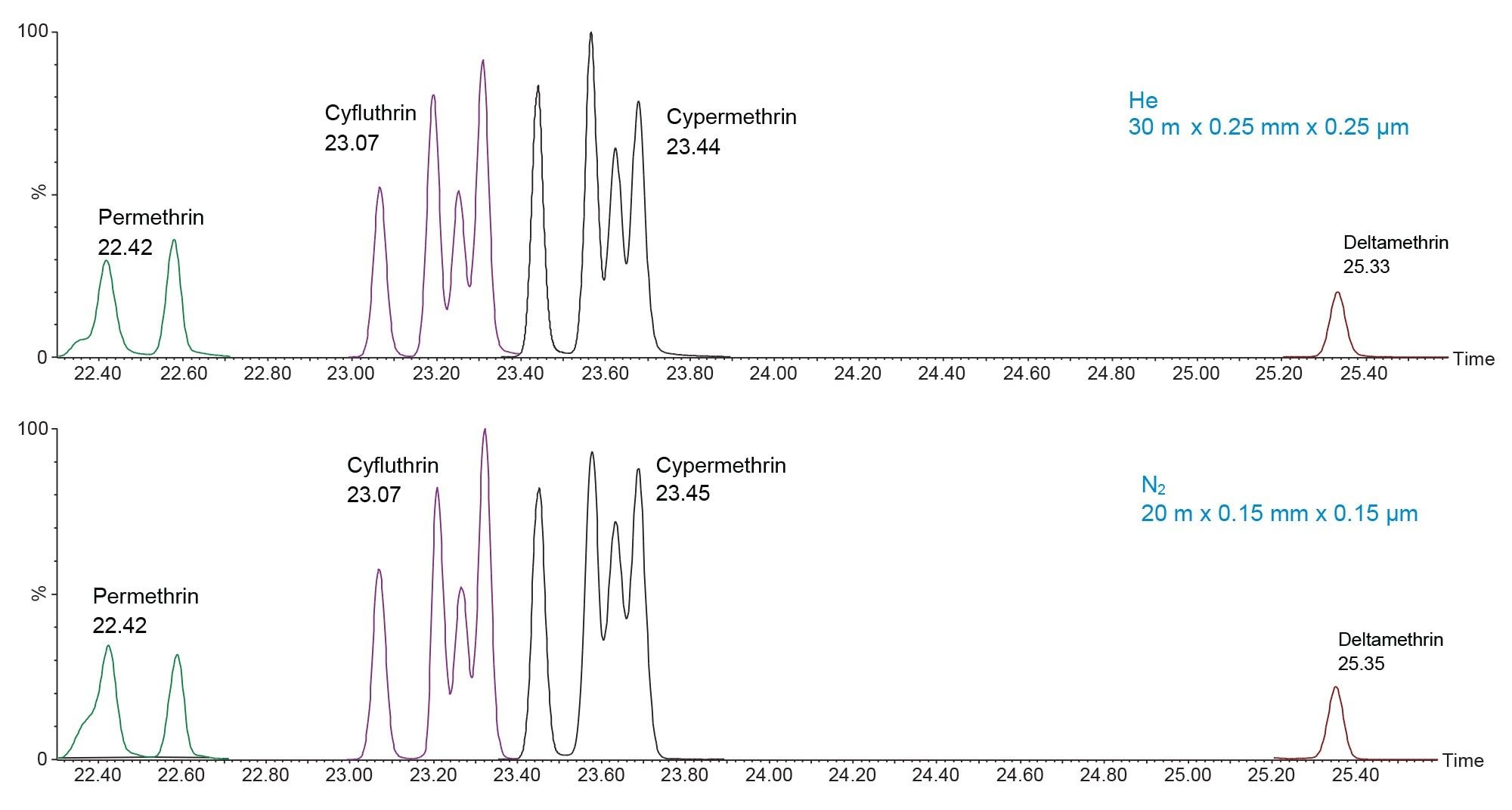
Figure 4 shows the chromatograms for the critical pair, profluralin (red), and terbutylazine (purple) where nitrogen carrier offers superior resolution. This could be due to the lower linear velocity of nitrogen giving each analyte slightly more time to interact with the stationary phase of the column.
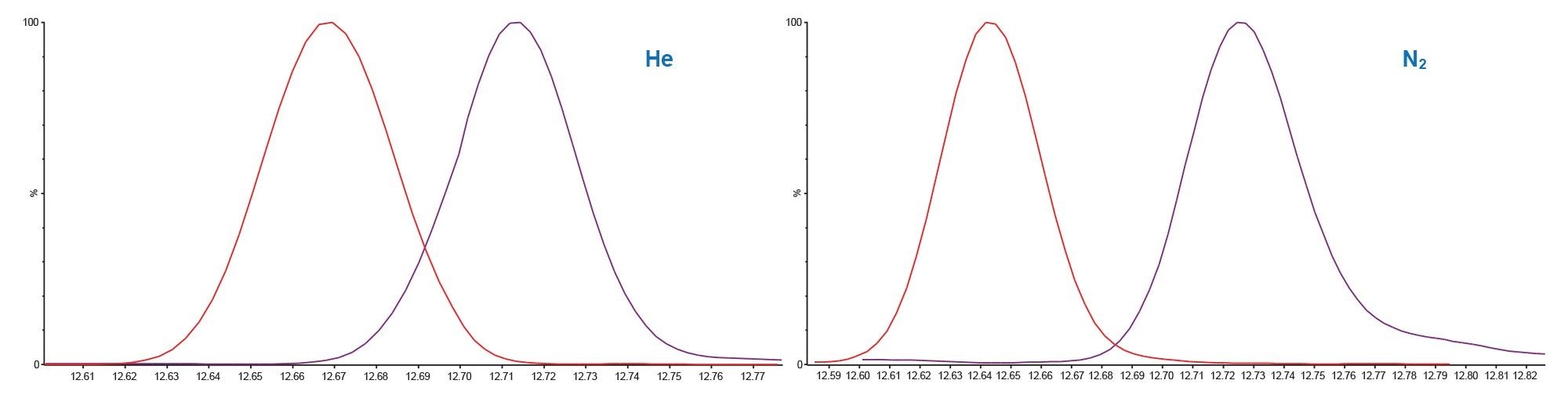
Repeatability
Each of the 6 internal standards used in this analysis returned residuals under 10% over 12 injections for each matrix. Figure 5 shows the comparison of an internal standard helium (left) and nitrogen (right) over multiple injections illustrating the residual plot with all injection falling within a 10% error range.
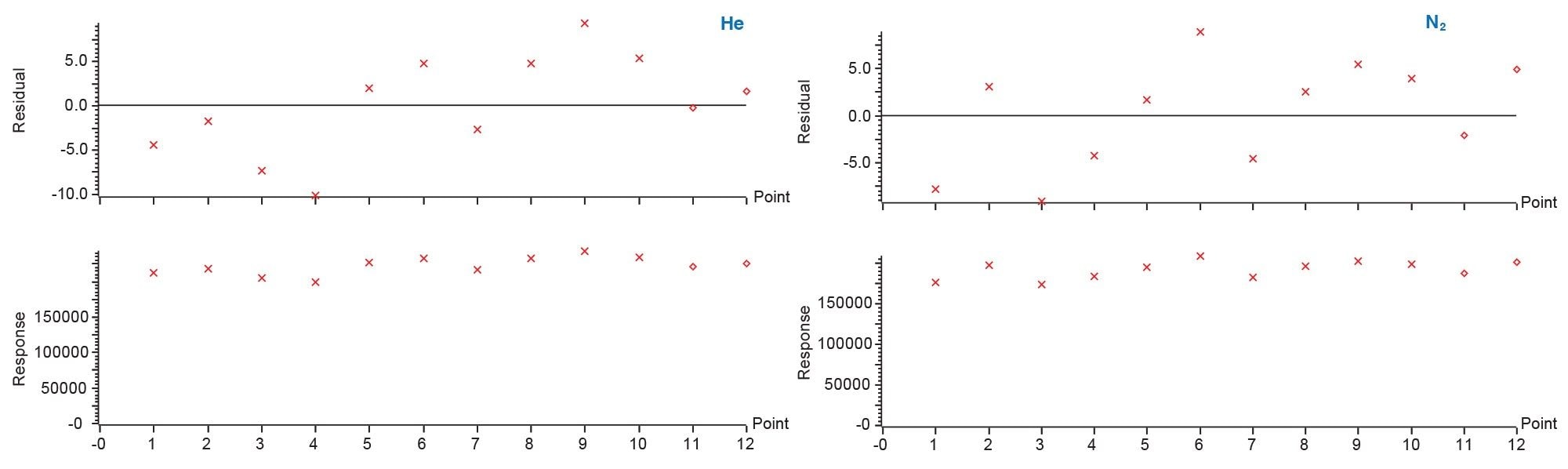
Sensitivity & Calibration Characteristics
The graph shown in Figure 6 shows the ratio of the helium response to the nitrogen response for the 0.01 mg/kg standard in infant food. This demonstrates the parity in sensitivity between the two carrier gases as approximately 59% of the analytes have a ratio between 0.5 & 1.5. 85% of the analytes give ratios less than 2.
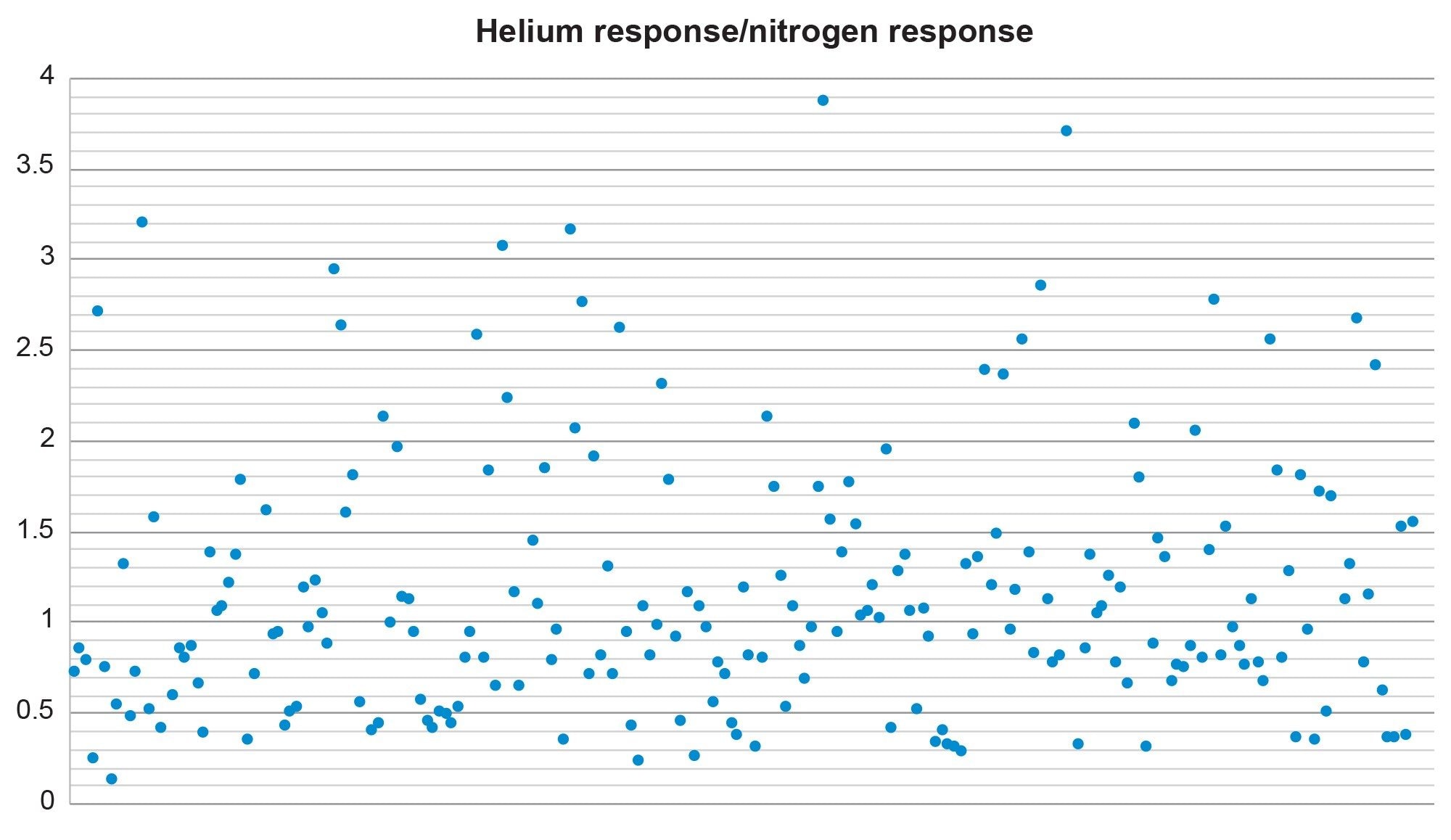
What follows are a list of historically “difficult” compounds detected at maximum residue level (MRL) 0.01 mg/kg all analyzed with nitrogen carrier, along with a comparison in sensitivity for cypermethrin and captan over each carrier gas. They all display residual plots within 20%, conforming to SANTE guidelines and R2 coefficients of >0.99.
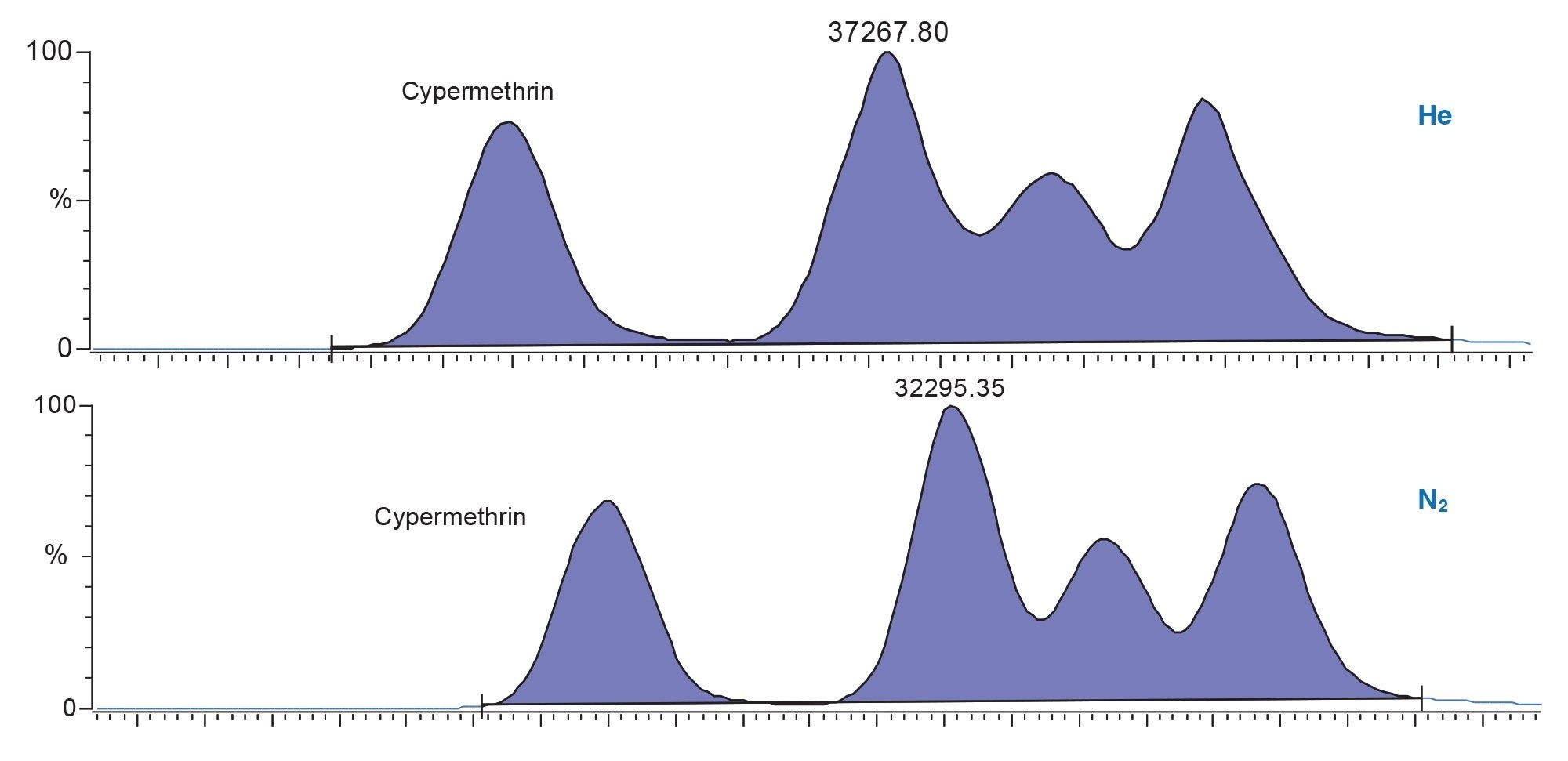
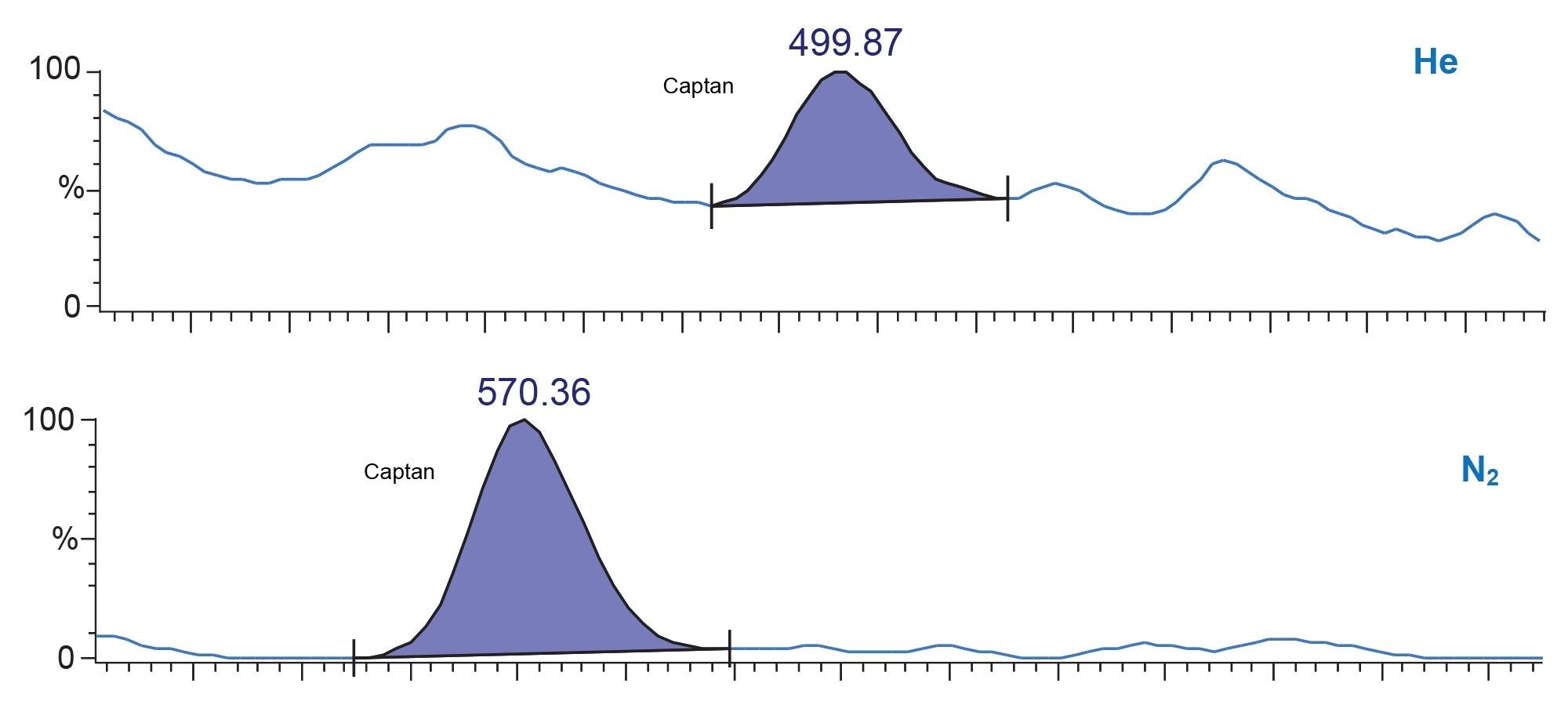
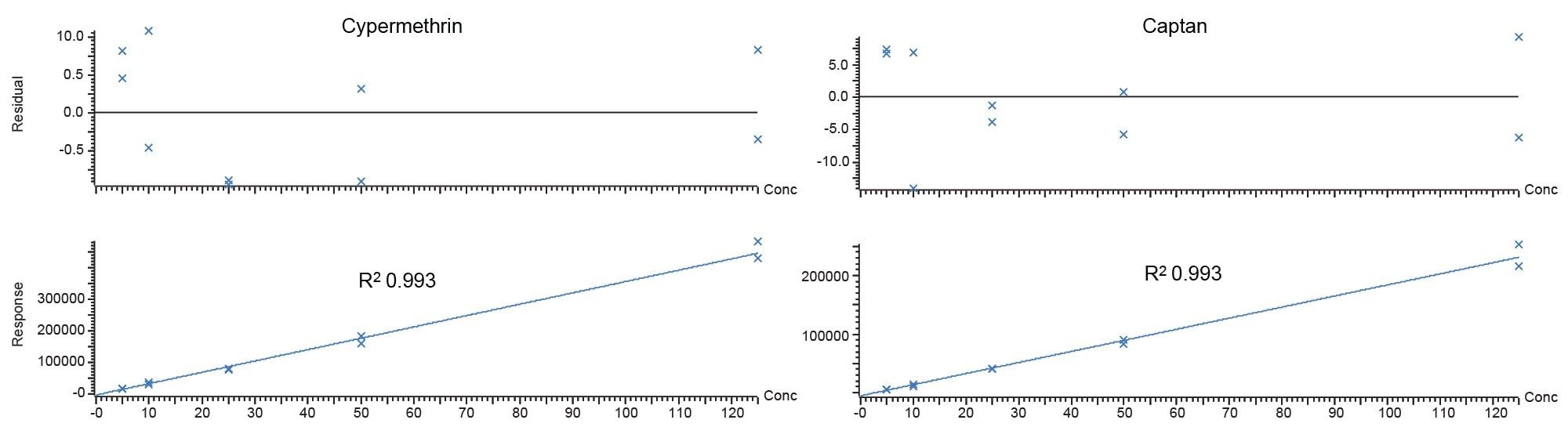
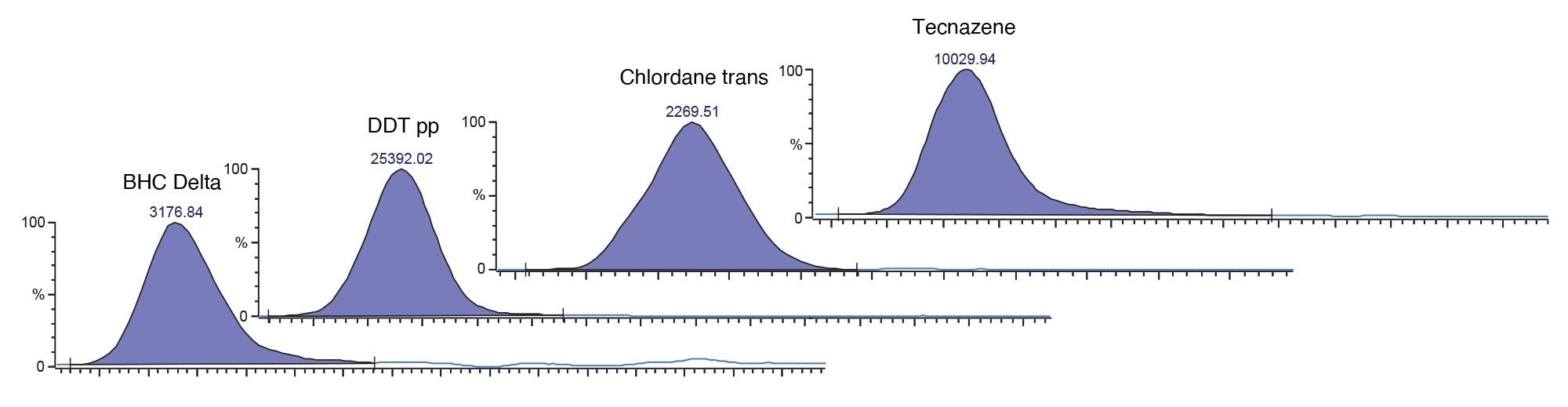
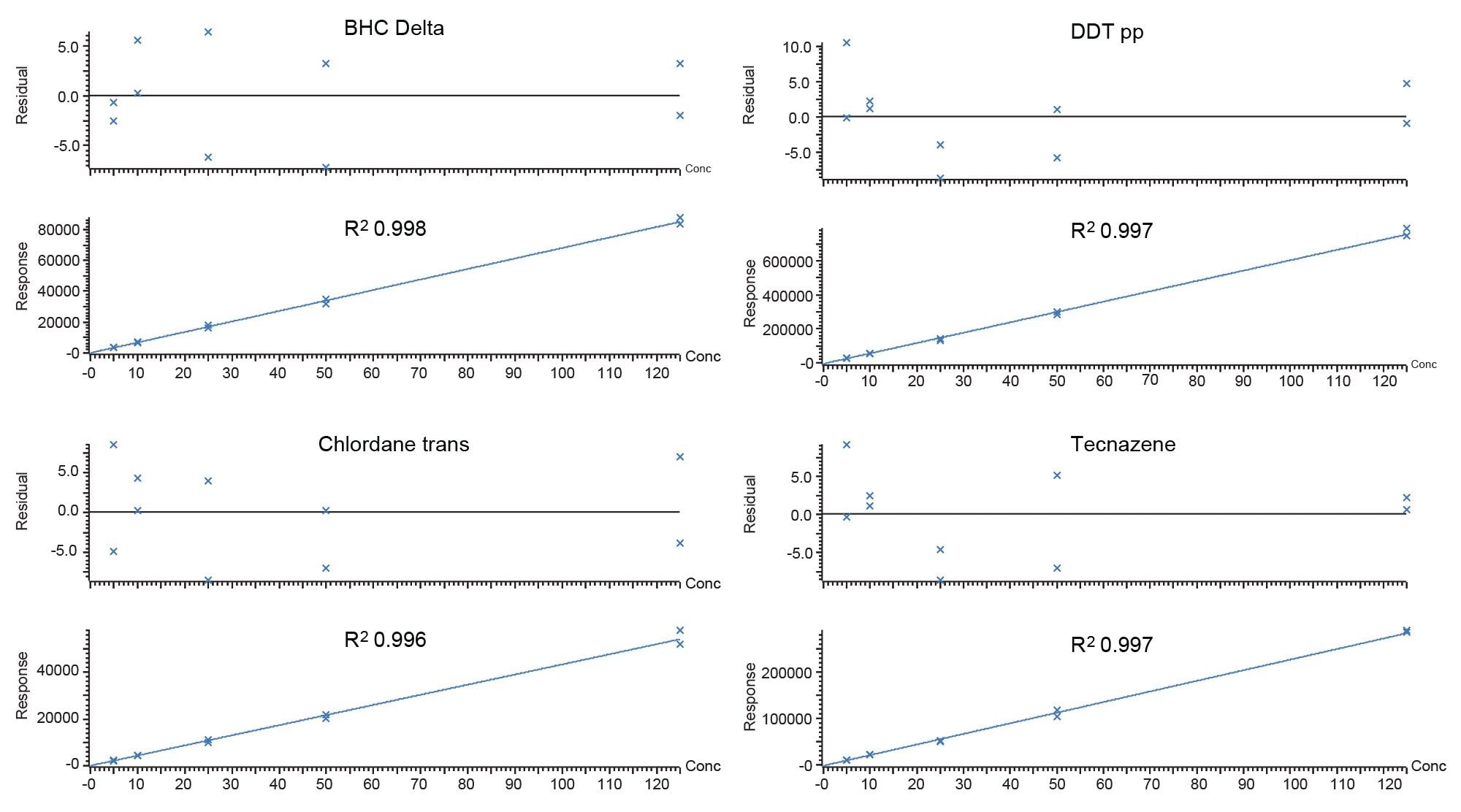
Conclusion
This application note describes the easy conversion of an existing GC method for the determination of pesticide residues from He to N2 as a carrier gas. As the ion source is not constrained by vacuum, the APGC system is able to switch to using N2 as a carrier gas without a loss in performance and can be returned to He at the turn of a valve. After scaling the column dimensions and adjustment of parameters, separations and run time were almost identical with no impact upon sensitivity. APGC with N2 as a carrier gas has been shown to be reliable for the determination of pesticide residues in food and offers considerable saving on carrier gas costs with no supply issues
References
- https://www.peakscientific.com/discover/news/how-is-helium-gas-obtained/.
- https://www.innovationnewsnetwork.com/helium-shortage-4-0-what-caused-it-and-when-will-it-end/29255/.
- European Committee for Standardisation (CEN) EN 15662:2018. Foods of Plant Origin - Multimethod for the Determination of Pesticide Residues Using GC- and LC- Based Analysis Following Acetonitrile Extraction/Partitioning and Clean-up by Dispersive Spe - Modular Quechers-Method.
- https://ez.restek.com/ezgc-mtfc.
720008044, September 2023