SYNAPT HDMS: Improving Ion Mobility Separation by Increasing Drift-Gas Polarizability
This is an Application Brief and does not contain a detailed Experimental section.
Abstract
In this technical note, we show that the polarizability and mass of the drift gas in the travelling-wave (T-Wave™) ion mobility region can have a marked effect on the separation of two butyl-aniline structural isomers.
Introduction
Ion mobility, and more recently SYNAPT™ HDMS™, has been used to analyze and separate a vast range of chemical entities that range from small molecules1 to polymers2, as well as large macromolecular protein complexes.3 However, in certain cases the separative power can be lacking on both the experimental drift tube instrumentation and the SYNAPT HDMS System. An attractive method increases the polarizability of the drift gas and the separative power of ion mobility. In this technical note, we demonstrate that drift gas polarizability increases the separation power of T-Wave ion mobility and enables the user to separate, identify, and characterize closely related structural isomers. As proof-of-principle examples, the two butyl-aniline structural isomers (Figure 1) were tested and differ only in theoretical collision cross-section (W) by 1 Å2 (based in helium ion mobility gas), as shown in Figure 1. Ion mobility separation, as a function of drift gas polarizability and molecular weight, has been demonstrated on an experimental drift tube instrument4 with halogenated aniline analogues; however, no research has ever been carried out on a T-Wave-based ion mobility system until now.
Different mono and polyatomic drift gases that range in polarizability and mass were tested to investigate T-Wave ion mobility separation on a SYNAPT HDMS System. The ion mobility drift gases used in this study were helium (He 4.0 mbar), argon (Ar 1.5 mbar), nitrogen (N2 1.5 mbar), carbon dioxide (CO2 1.5 mbar), and ethene (C2H4 1.5 mbar). The recorded gas pressure are pirani gauge indicated pressures, not absolute pressure measurements.
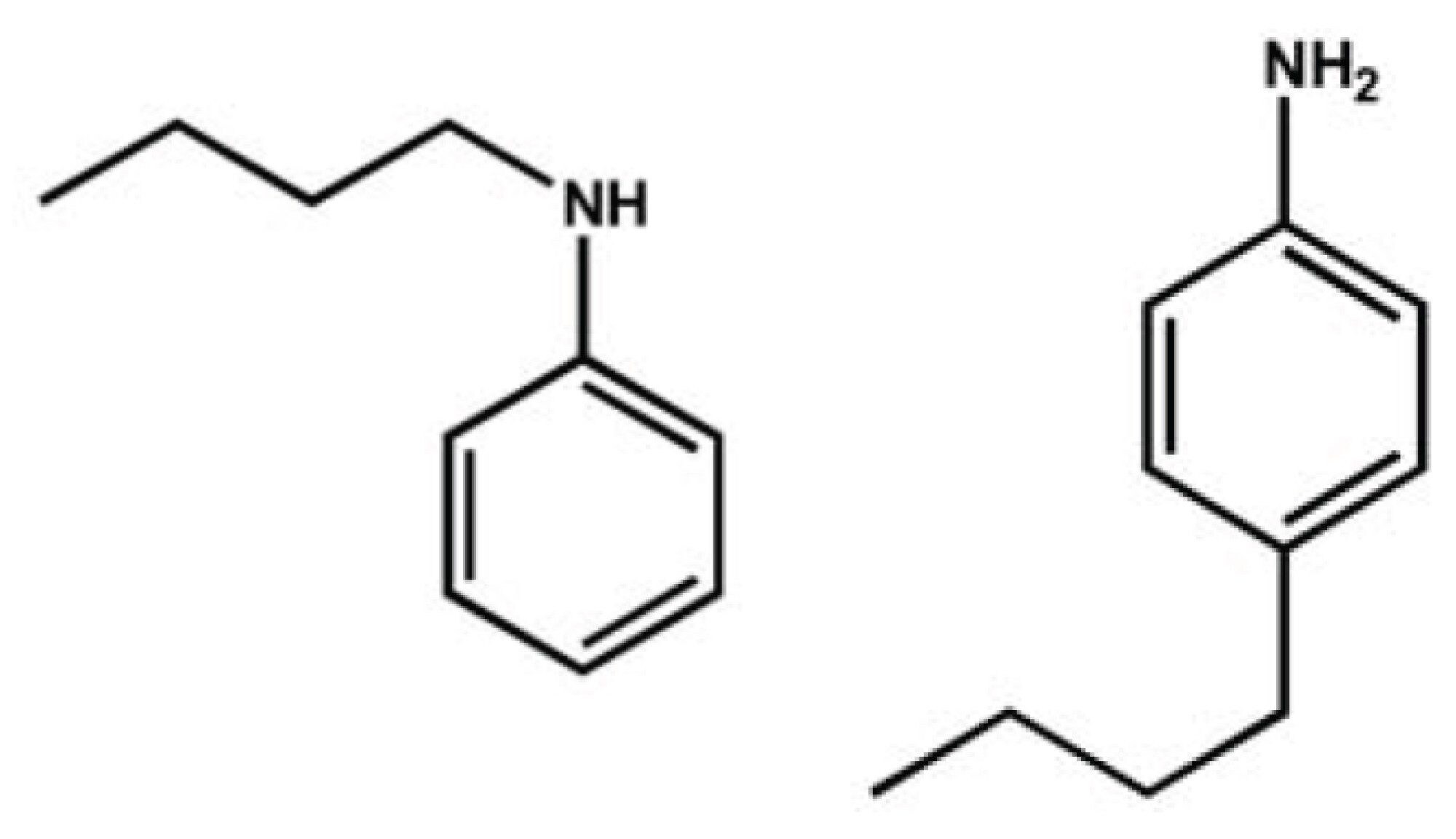
Experimental
MS Conditions
MS system: |
SYNAPT HDMS |
IMS: |
He, Ar, N2, CO2, and C2H4 1.5 to 4.0 mbar pulse height 4.5 to 30.0 V (depending on which drift gas was used), 250 m/sec |
Trap/transfer: |
2.0 e-2 mbar SF6 |
T-Wave ion mobility calibration was performed utilizing a previously documented procedure.5 This procedure used calibration standards polyalaline and polyglycine, whose W values have previously been determined utilizing a drift tube instrument.6
Alkyl-substituted anilines, shown in Figure 1, represent a family of isomers that has challenged most MS approaches for isomer recognition and resolution.1 Their EI-MS, CI-MS(/MS), and ESI-MS(/MS)1 results are usually very similar and fail to distinguish these important constitutional isomers. Here, we describe how a combination of theoretical Ω determination, T-Wave ion mobility and the use of more polar drift gases can be used to routinely distinguish a pair of butyl-substituted aniline structural isomers.
The theoretical collision cross-sections (W) calculated using the MOBCAL Trajectory Method8 for N-butylaniline and 4-butylaniline are 76.6 Å2 and 77.6 Å2 respectively (based in helium). This suggests the N-butylaniline isomer is the more mobile species and therefore, shows a shorter ion mobility drift time.
Figure 2 demonstrates that the N-butylaniline isomer is in fact more mobile. This is in agreement with the theoretical MOBCAL prediction.
Figure 2 also shows the effect of increasing the mass (and size) and polarizability of the drift gas. The individual drift gas masses and polarizability constants are noted in Table 1. It is clearly observed that the use of more massive, polar-polyatomic drift gases, such as carbon dioxide and ethylene, resulted in improved T-Wave ion mobility separation; where helium, the lightest and least polarizable gas resulted in very little T-Wave ion mobility separation.
This improved T-Wave ion mobility separative power afforded by different drift gases has far reaching analytical implications, such as its application in the separation of small molecule metabolites, Chinese herbal medicines, and organometallic synthesis. For example, in a recent publication5, researchers were able to separate an ortho- and para-terphenyl ruthenium anticancer drug to 50% valley using nitrogen as the ion mobility drift gas. If one were to use carbon dioxide, or ethene, a significant improvement in ion mobility separation would be achieved, thus aiding structural isomer characterization.
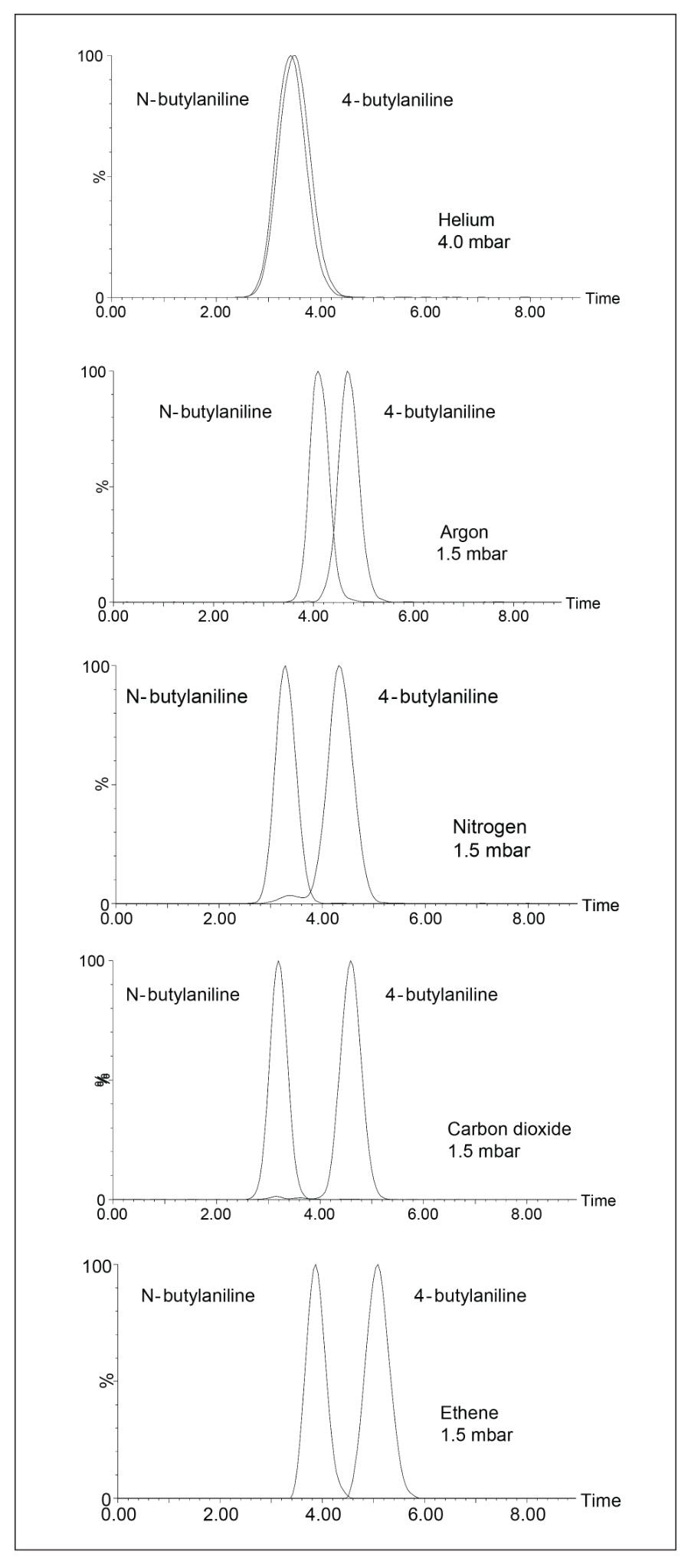
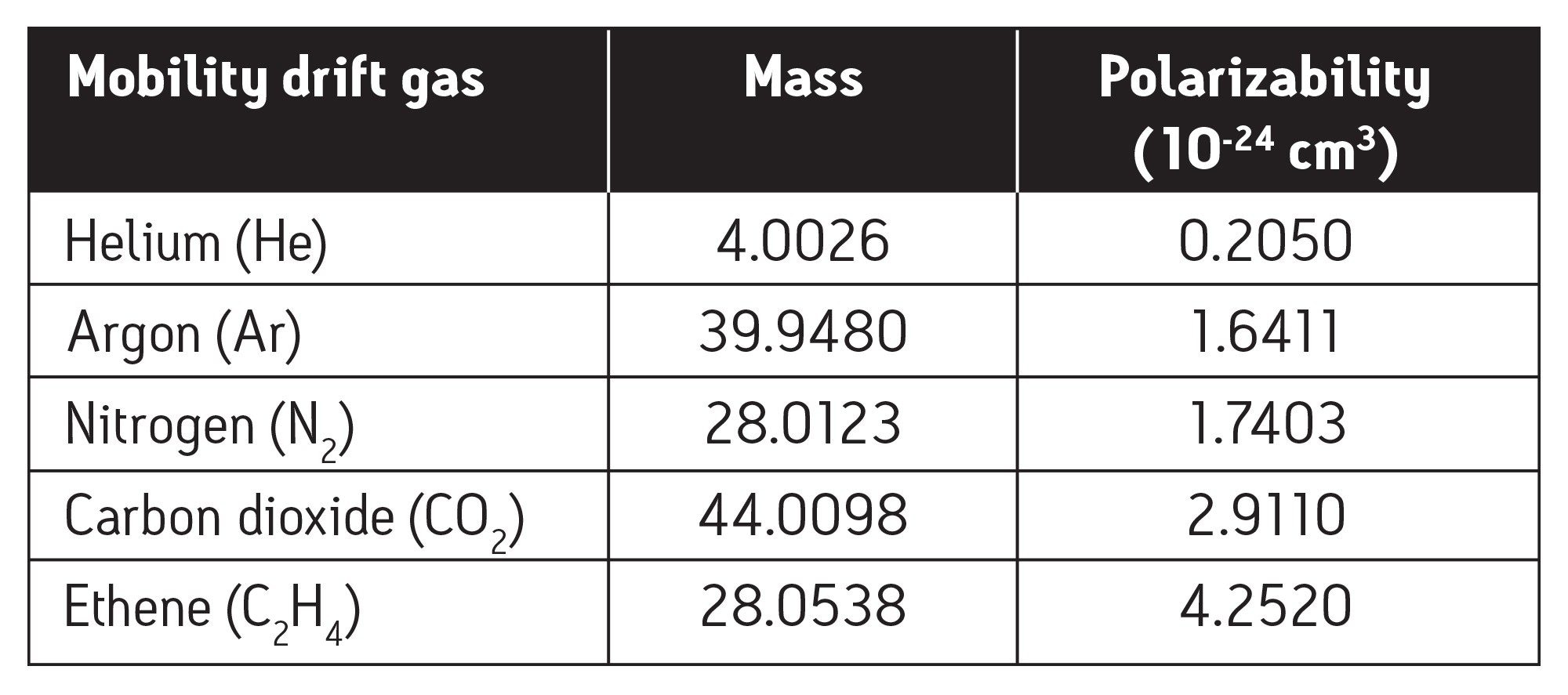
Conclusion
The T-Wave ion mobility separation capability of the SYNAPT HDMS System was investigated as a function of drift gas polarizability and mass.
We have demonstrated that significantly different T-Wave ion mobility separation of two small butyl-aniline structural isomers can be achieved using more massive and polarizable drift gases, such as carbon dioxide and ethene.
T-Wave ion mobility baseline separation was achieved on two butyl-aniline structural isomers using carbon dioxide as the ion mobility drift gas.
References
- Benassi et al. J Am Soc Mass Spectrom. 2009, 20, 269–277.
- Bagal et al. Anal Chem. 2008, 80, 2408–2418.
- Ruotolo et al. Science. 9th December 2005, 310, 1651–1661.
- Asbury & Hill Anal Chem. 2000, 72, 560–584.
- Williams et al. J Am Soc Mass Spectrom. 2009, 20 (6) 1119–1122.
- http://www.indiana.edu/~clemmer/
- Lide et al. CRC Handbook of Chemistry and Physics, 70th ed. CRC Press: Boca Raton, FL, 1989.
- Mesleh et al. J Phys Chem. 1996, 100, 16082–16086.
720003201, August 2022